Free Energy
We only talk about free energy when it changes (ΔG). Much like how you only talk about your friends when they aren't listening. Basically, ΔG describes the change in useful energy that can be extracted from a particular reaction to do work. Let’s rewrite the Gibbs equation in terms of ΔG:
ΔG = ΔH - TΔS.
Now the Gibbs equation says that the amount of useful, or free, energy (ΔG) that can be extracted from a reaction equals the total energy change (ΔH) minus the energy that cannot do work because it increased the disorder of the system (TΔS). Make a little more sense? Good.
Why is solving for ΔG useful? ΔG tells us whether a reaction will proceed or not, which is a nice thing to know.
This process of hooking together is called coupling, and your cells use coupling all the time to perform many of the energetically unfavorable reactions in a cell. We can think of coupling as using the energy gained from a downhill reaction (a spontaneous and favorable one), to help power an uphill reaction (a nonspontaneous and energy-needy one).
What happens when ΔG = 0? This type of reaction is considered to be at equilibrium, and therefore, there is no net change of energy going in or out of the system. We will go into the ins and outs of achieving equilibrium a little later. The major take-home message for understanding the Gibbs equation is that it lets you predict the outcome of a reaction. Think of it as ESP in the thermodynamic dimension.
If you are having a hard time distinguishing the difference between the change in entropy (TΔS) and the change in free energy (ΔG), this paragraph is for you. At first, it may seem confusing. Contradictory even. A reaction that increases the disorder in a system is said to increase the entropy. You already know that the universe is relentlessly moving toward greater entropy. Your Spidey sense is right if you think that a reaction that causes a system to become extremely disordered (positive TΔS) is likely to be spontaneous (negative ΔG—dangerous!). Unfortunately, knowing only the change in energy in a system or knowing only the change in entropy in a system is not enough to predict whether a reaction will occur spontaneously. You need to know both factors. This need is what makes free energy such an important factor in the equation. Hehe. A reaction with a negative ΔG always occurs spontaneously.
Time to think about making popsicles. Never done it? You are missing out. You pour some juice into a mold, and freeze it. Easy as pie. Actually, way easier than pie. Whoever came up with that idiom had obviously never made popsicles. Freezing the juice decreases the entropy of the system because the molecules become more rigid and in an ordered state. For juice to freeze, a large amount of heat needs to be released from the system. When do you think that the popsicles will freeze? If you said "when you put it in the freezer," congratulations, you win! But, why will the popsicles freeze only at temperatures below 0 °C? Because, only at temperatures below 0 °C will ΔH be negative enough to compensate for the negative TΔS value.
Here is a table to make your life a tiny bit easier.
What? We did say "tiny bit."
Now that your head is full of Hs, Gs, Ss, and Ts (HGST!), we will finally look at some biology, specifically, cellular reactions from a thermodynamic perspective.
First, know that all reactions are referred to as having directions: forward, neutral, or backward.
Second, know that all biochemical reactions are reversible even if one direction of the reversible reaction rarely occurs.
We like to refer to these reactions as the forward reaction and the reverse reaction, with reactants (that…react) on one side of the reaction equation, and products (that are…produced) on the other side of the reaction equation.
Important: If the ΔG of the forward reaction is negative, the reaction will proceed spontaneously forward in the direction of the products.
Example Reactions
A + B ⇒ AB
A and B are reactants, and AB is the product. This reaction proceeds forward (double arrow).
A + B ⇔ C+ D
A and B are reactants, and C and D are products, OR C and D are reactants, and A and B are products (two-way arrow).
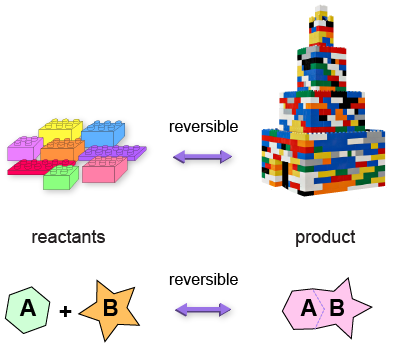
We know; we lied. We said that this would be all physics and no chemistry. Honestly, it's not our fault that everything in the universe is so well connected.
These tidbits are all fine and dandy, but (there is always a "but") knowing that a reaction is spontaneous is not enough to gauge how fast a reaction will occur. Sure, a reaction with a negative ΔG will occur, but it might be hundreds of years until you see any noticeable amount of product. If you think back to our earlier example, it would be like your parents saying that they will pay your allowance…sometime. Timing is pretty darn important.
What determines how fast a reaction will occur? (We refer to this as the rate of the reaction.)
There are two factors we will discuss:
One important factor is the concentration of the reactants and products. In fact, chemical reactions will proceed until they reach equilibrium, or the point where the forward and reverse reactions are occurring at the same rate. At equilibrium, the amount of reactants used to make the product is the same as the amount of reactants produced from the product. That is, the forward reaction rate equals the reverse reaction rate. In other other words, at equilibrium, there is a constant ratio of reactants to products. Examples are 1:1, 2:1, and so on.
Equilibrium likes to make sure that sharing is at 100% and that there are no squabbles. Equilibrium is an awesome pie-slicer.
Temperature
Another important factor for determining the reaction rate is the temperature of the reaction. It turns out that most of the reactions in your body require surprisingly high temperatures to occur quickly—much higher than your body temperature of 37 degrees Celsius or 98.6 degrees Fahrenheit. What? How can that be possible?
Enzymes are the answer (finally, some biology). Enzymes are usually proteins, but not any old run-of-the-mill protein earns the enzyme label. Enzymes catalyze, or accelerate, reactions. Unlike reactants and products, which are either directly used or produced in a chemical reaction, enzymes change the rate the reaction but are not consumed or produced in the reaction.
Enzymes are conservative little fellows that won't change for anybody or anything. Enzymes will not change based on the thermodynamics of a reaction. They won’t add energy, entropy, or enthalpy, or temperature changes to a reaction, and they absolutely will not determine whether a reaction is thermodynamically favorable or not. They get down to bizness and make reactions occur much faster. Enzymes are the focus of our next section.
Well, that's a wrap for us on free energy. You have accomplished quite a lot by tolerating the physics presented here: by understanding thermodynamics, you will now be able to understand the biology of the cell on a whole new and improved level. Energy is not only what makes a cell run smoothly, but it also governs the basics of cell morphology (shape) and cellular biochemical reactions. If you so desired, you could look at all biological structures from an energy perspective. Many of the "Why?" questions in biology can be answered by gaining a better understanding of thermodynamics. For example, "Why does a plasma membrane behave the way it does?" Answer: Check out the Big Theme section in this unit on structure and function.
Brain Snack
What does the word "enzyme" mean? Its origin is Greek, and it means "in yeast." In the late 1800s, there was a debate about how alcohol was produced from yeast. Louis Pasteur thought that there was something special about the yeast that allowed the reaction to occur. In 1897, the Buchner brothers ground yeast cells and showed that Pasteur was right—there was something special biological molecules in the yeast cells that promoted fermentation: enzymes. You'll probably appreciate that more in a few years.
ΔG = ΔH - TΔS.
Now the Gibbs equation says that the amount of useful, or free, energy (ΔG) that can be extracted from a reaction equals the total energy change (ΔH) minus the energy that cannot do work because it increased the disorder of the system (TΔS). Make a little more sense? Good.
Why is solving for ΔG useful? ΔG tells us whether a reaction will proceed or not, which is a nice thing to know.
- If ΔG is negative, the reaction is said to be exergonic, meaning that the process will occur spontaneously and is thermodynamically favorable. Heat will often be released, which in turn creates disorder.
- If ΔG is positive, then the reaction is said to be endergonic, meaning that it is thermodynamically unfavorable. In other words, this type of reaction needs the input of energy to make it happen.
This process of hooking together is called coupling, and your cells use coupling all the time to perform many of the energetically unfavorable reactions in a cell. We can think of coupling as using the energy gained from a downhill reaction (a spontaneous and favorable one), to help power an uphill reaction (a nonspontaneous and energy-needy one).
What happens when ΔG = 0? This type of reaction is considered to be at equilibrium, and therefore, there is no net change of energy going in or out of the system. We will go into the ins and outs of achieving equilibrium a little later. The major take-home message for understanding the Gibbs equation is that it lets you predict the outcome of a reaction. Think of it as ESP in the thermodynamic dimension.
If you are having a hard time distinguishing the difference between the change in entropy (TΔS) and the change in free energy (ΔG), this paragraph is for you. At first, it may seem confusing. Contradictory even. A reaction that increases the disorder in a system is said to increase the entropy. You already know that the universe is relentlessly moving toward greater entropy. Your Spidey sense is right if you think that a reaction that causes a system to become extremely disordered (positive TΔS) is likely to be spontaneous (negative ΔG—dangerous!). Unfortunately, knowing only the change in energy in a system or knowing only the change in entropy in a system is not enough to predict whether a reaction will occur spontaneously. You need to know both factors. This need is what makes free energy such an important factor in the equation. Hehe. A reaction with a negative ΔG always occurs spontaneously.
Time to think about making popsicles. Never done it? You are missing out. You pour some juice into a mold, and freeze it. Easy as pie. Actually, way easier than pie. Whoever came up with that idiom had obviously never made popsicles. Freezing the juice decreases the entropy of the system because the molecules become more rigid and in an ordered state. For juice to freeze, a large amount of heat needs to be released from the system. When do you think that the popsicles will freeze? If you said "when you put it in the freezer," congratulations, you win! But, why will the popsicles freeze only at temperatures below 0 °C? Because, only at temperatures below 0 °C will ΔH be negative enough to compensate for the negative TΔS value.
Here is a table to make your life a tiny bit easier.
Type of Reaction | Spontaneous Reaction? | Free Energy Change (Δ G) | Entropy Change (T Δ S) | Enthalpy Change (Δ H) |
---|---|---|---|---|
Exergonic | Yes | Negative, and Δ G < 0. | Positive, or increasing, and T Δ S 0. | System may absorb or release heat, so Δ H > 0 OR Δ H < 0. If Δ H > 0, then Δ H < T Δ S . |
Exergonic | Yes | Negative, and Δ G < 0. | Negative, or decreasing, and T Δ S < 0. | System must release heat, so Δ H < 0, and |Δ H > | T Δ S |. |
Endergonic | No | Positive, and Δ G > 0. | Positive, or increasing, and T Δ S > 0. | System must absorb heat, so Δ H > 0, and Δ H > T Δ S . |
Endergonic | No | Positive, and Δ G > 0. | Negative, or decreasing, and T Δ S < 0. | System may absorb or release heat, so Δ H > 0 OR Δ H < 0. If Δ H < 0, then |Δ H< | TΔ S |. |
Now that your head is full of Hs, Gs, Ss, and Ts (HGST!), we will finally look at some biology, specifically, cellular reactions from a thermodynamic perspective.
First, know that all reactions are referred to as having directions: forward, neutral, or backward.
Second, know that all biochemical reactions are reversible even if one direction of the reversible reaction rarely occurs.
We like to refer to these reactions as the forward reaction and the reverse reaction, with reactants (that…react) on one side of the reaction equation, and products (that are…produced) on the other side of the reaction equation.
Important: If the ΔG of the forward reaction is negative, the reaction will proceed spontaneously forward in the direction of the products.
Example Reactions
A + B ⇒ AB
A and B are reactants, and AB is the product. This reaction proceeds forward (double arrow).
A + B ⇔ C+ D
A and B are reactants, and C and D are products, OR C and D are reactants, and A and B are products (two-way arrow).
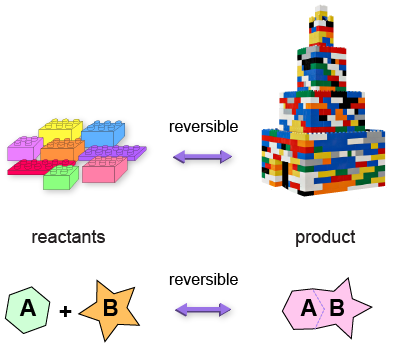
We know; we lied. We said that this would be all physics and no chemistry. Honestly, it's not our fault that everything in the universe is so well connected.
These tidbits are all fine and dandy, but (there is always a "but") knowing that a reaction is spontaneous is not enough to gauge how fast a reaction will occur. Sure, a reaction with a negative ΔG will occur, but it might be hundreds of years until you see any noticeable amount of product. If you think back to our earlier example, it would be like your parents saying that they will pay your allowance…sometime. Timing is pretty darn important.
What determines how fast a reaction will occur? (We refer to this as the rate of the reaction.)
There are two factors we will discuss:
- Equilibrium
- Temperature
One important factor is the concentration of the reactants and products. In fact, chemical reactions will proceed until they reach equilibrium, or the point where the forward and reverse reactions are occurring at the same rate. At equilibrium, the amount of reactants used to make the product is the same as the amount of reactants produced from the product. That is, the forward reaction rate equals the reverse reaction rate. In other other words, at equilibrium, there is a constant ratio of reactants to products. Examples are 1:1, 2:1, and so on.
Equilibrium likes to make sure that sharing is at 100% and that there are no squabbles. Equilibrium is an awesome pie-slicer.
Temperature
Another important factor for determining the reaction rate is the temperature of the reaction. It turns out that most of the reactions in your body require surprisingly high temperatures to occur quickly—much higher than your body temperature of 37 degrees Celsius or 98.6 degrees Fahrenheit. What? How can that be possible?
Enzymes are the answer (finally, some biology). Enzymes are usually proteins, but not any old run-of-the-mill protein earns the enzyme label. Enzymes catalyze, or accelerate, reactions. Unlike reactants and products, which are either directly used or produced in a chemical reaction, enzymes change the rate the reaction but are not consumed or produced in the reaction.
Enzymes are conservative little fellows that won't change for anybody or anything. Enzymes will not change based on the thermodynamics of a reaction. They won’t add energy, entropy, or enthalpy, or temperature changes to a reaction, and they absolutely will not determine whether a reaction is thermodynamically favorable or not. They get down to bizness and make reactions occur much faster. Enzymes are the focus of our next section.
Well, that's a wrap for us on free energy. You have accomplished quite a lot by tolerating the physics presented here: by understanding thermodynamics, you will now be able to understand the biology of the cell on a whole new and improved level. Energy is not only what makes a cell run smoothly, but it also governs the basics of cell morphology (shape) and cellular biochemical reactions. If you so desired, you could look at all biological structures from an energy perspective. Many of the "Why?" questions in biology can be answered by gaining a better understanding of thermodynamics. For example, "Why does a plasma membrane behave the way it does?" Answer: Check out the Big Theme section in this unit on structure and function.
Brain Snack
What does the word "enzyme" mean? Its origin is Greek, and it means "in yeast." In the late 1800s, there was a debate about how alcohol was produced from yeast. Louis Pasteur thought that there was something special about the yeast that allowed the reaction to occur. In 1897, the Buchner brothers ground yeast cells and showed that Pasteur was right—there was something special biological molecules in the yeast cells that promoted fermentation: enzymes. You'll probably appreciate that more in a few years.