The Immune System
Kicking the Bad Guys Out
It's payday. You're standing in line at the bank, minding your own business and finally cashing in on your meager paycheck. Maybe you'll buy a snazzy jacket with glittery buttons. You hear the quiet din of the soothing bank music mixed with conversation. You wonder if it might be faster to just use an ATM.Something is off. You see three Jesse James wannabes in cowboy hats and spurs, and they're yelling at you to get down on the floor. The bank teller, also wearing a sweet cowboy hat (wait, when are we again?), pulls out a revolver and foils the robbers' plan. The snazzy jacket is saved.
With just a few minor tweaks, this foiled bank heist could be the drama that takes place within our body's immune system. Basically, when an intruder—pathogen is the million dollar word—makes its way into the body, a group of cells recognize the oddball in the ski mask and attacks it. Maybe not with a six-shooter, but the immune system is killing the pathogens to keep all things inside you happy and healthy.
All animals, from the lowly fruit fly to us Snapchatting humans, have immune systems. We’re going to focus on the vertebrates, and specifically the mammals, but that doesn’t mean invertebrates are defenseless.
Since the immune system needs to function all over the body (microbes can enter pretty much anywhere), immune organs are located throughout the body. Collectively, they are called lymphoid organs. In animals with bones, every bone contains bone marrow, and this is the place where all immune cells are born as stem cells. In vertebrates without true bones, like cartilaginous fish (think sharks and rays), immune cells are made in the spleen and kidneys.
Regardless of where the immune cells originate, some of them will stay home to develop, while others travel to distant lands, like the thymus, which is located directly under the breast bone in mammals. Each animal also has a spleen and lymph nodes (located in the neck, armpit, abdomen, and groin for mammals) where immune cells congregate to combat incoming pathogens. Think of them as military bases where the army can gear up before going into battle.
All of these lymphatic organs are connected with a lymphatic network of vessels. These vessels line up with blood vessels, and there's a constant exchange between the two, so the immune system can immediately recognize any strange-looking invaders that are hitching a ride through the blood vessels.
From here on out, we’ll just be talking about mammals, to cut down on the number of awkward asides. Mammals have two types of immune systems to keep them going:
- The innate immune system provides a general defense strategy for combating and keeping out pathogens.
- The adaptive immune system responds more systematically to specific threats that might make it over the wall and into the body.
Innate Immune System
The first line of defense is provided by an organism's innate immunity system. All animals have one, and it's made up of all the immediate reactions their bodies have to a pathogen. If we return to our handy bank metaphor, the revolving doors are the innate immune system, generally trying to keep bad stuff outside, whether it be robbers, cold winds, or irate giraffes.Pathogens looking to wreak havoc in the body first come across a barrier defense—skin, tears, long white nose hairs—which make it hard for things that shouldn't be in the body to make their way inside. If these pathogens make it past the formidable barrier opponents, the body's internal innate defensive line jumps into action.
The body increases production of white blood cells, or leukocytes, that launch an attack on the invading pathogens. If necessary, an animal will develop a fever to combat the growth of bacteria at this point, too. They may make you feel cruddy, but fevers are your body fighting the good fight. Sometimes they even get you out of school. Fevers are the best.
Leukocytes
All leukocytes come from the bone marrow, but the way they ward off the bad guys differs. Some leukocytes carry out phagocytosis (and are called phagocytes), where they go all dinosaur on pathogens, straight up swallowing and devouring them. There are several different types of leukocytes in the innate immune system, but they generally fit into the following categories:- Macrophages: Vertebrates are lucky and have some effective phagocytes called macrophages that are super hungry for pathogens. Consider them the T-Rex of the lot.
- Granulocytes: These cells have small pockets, or granules, with potent killer-chemicals inside. When a granulocyte swallows a virus or protozoa, nasty chemicals are released from these granules to kill the invader.
- Natural Killer Cells: The name says it all. These cells are hard-core pathogen destroyers, the AK-47s of immunity. These cells are so die-hard they kill virally infected cells on contact, without the need for digestion or internal granules.
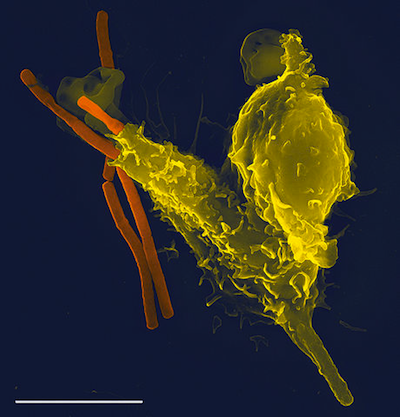
Phagocytosis of anthrax bacteria. A super-zoomed in picture of a leukocyte (yellow sphere) consuming the anthrax bacteria (orange rod). Image from here.
The innate immune system is completely indebted to phagocytes. Not only do they have a fond taste for pathogens, but they also secrete communication chemicals called cytokines. These chemicals tell other cells (other phagocytes and cells in the adaptive immune system) that they should get their act together and prepare for an attack.
When Infection Strikes
When cells become virally infected, they release interferons that alert neighboring innate immune cells of the unwelcome guest. It's like a burglar alarm going off in a sleepy neighborhood. Neighboring cells then prepare themselves for attack (maybe they'll dead bolt their doors or wake up their ill-tempered Rottweiler), and secrete chemicals that prevent the virus from spreading.All of these cells and chemicals of the innate system have a common purpose: ward off infection. This system has figured out some of the best ways to do just that.
If pathogens enter the body through the skin (like if you stepped on a rusty nail, for example), the innate immune system launches an inflammatory response to kill the invading pathogens. Inflammation is simply an attempt at isolating infection or trauma, and preventing it from affecting the rest of the body. Increased blood flow is why the damaged area is red and feels hot. All that extra blood brings antimicrobial proteins that attract more macrophages and bump up the pathogen-killing power even more.
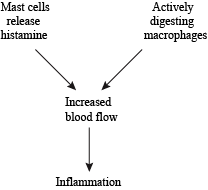
Inflammation—Since it's such an important part of the immune system, there are lots of players that contribute to the inflammatory process.
Another part of the innate immune system is involved in tissue repair. Phagocytes are the key players here, since they devour dead or damaged cells. Once the accident has been cleaned up and all is functioning again, certain chemicals will stimulate new tissue growth and get things back to normal.
With all the macrophages working overtime and consuming dangerous pathogens, they also secrete toxins that affect body temperature and cause a fever. It's just another step in this world of the innate immune response.
Adaptive Immune System
The innate immune system typically does a great job with its wide range of tools that it sets loose on an intruder. Just to be on the safe side, though, vertebrates have a second line of defense that only gets turned on if the pathogen somehow survived the innate immune system. This adaptive immune system responds to specific pathogens. It's like a security guard with a laser sight that only takes aim at specific bank robbers, like those named Bill, or Simon, or Cholera.The immune cells in this specialized immune system get a special name: lymphocytes. These white blood cells are divided into two groups: the humoral and cell-mediated immune systems.
The humoral immune system is composed of B cells, which begin their life as stem cells in the bone marrow. Once B cells are formed, they migrate to the spleen and lymph nodes to continue their development.
B cells are activated by antigens, which are the biological trademarks of pathogens. These are usually proteins, and they can be a part of the pathogen's cell wall or flagella. Each type of bacteria or protozoa has at least one distinct antigen, meaning there are countless antigens out there in the big wide world.
B cells have receptors that are extremely picky when it comes to finding their antigen of choice, and can distinguish one from another with incredible accuracy. They aren't content to lay waste to just any old pathogen. No sir, each one of these B cells is looking for their long-lost nemesis, which they have been trained to hunt and destroy. For these lymphocytes, it's personal.
Antigens are moving around through the lymph, and when a B cell finds its matching foreign antigen, it does two things: makes antibodies and makes daughter cells. An antibody is a water-soluble form of the B-cell receptor, and it can go places where B cells can't. Their purpose is the same: to track down and tag pathogens for demise. B-cell receptors are bound to their cell membranes, while the antibodies that get secreted are free to move around the body.
B cells love their antibodies; that's why they make lots of them super-fast—on the order of 2000 antibodies each second for as long as the B cell is activated (usually around 4 days). That's a lot of antibodies: 691,200,000 to be exact (with this estimate).
Antibodies have a "Y" shape and can bind antigens with both arms. Like B-cell receptors, each antibody is designed to recognize a particular antigen, and their "Y" arms have chemical properties that distinguish between different antigens.
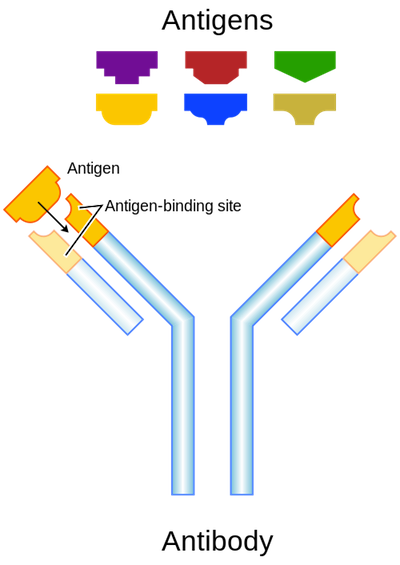
Antibodies bind with high specificity to their particular antigens. Image from here.
Just like the B-cell receptor, antibodies recognize a particular antigen in the body fluids, bind to it (they fit together like a lock and key), and tag it for demise. The antibody binds to the pathogen cell surface, disrupting the intruder’s ability to harm other cells.
For macrophages, antibodies act like the sprinkles and chocolate syrup on a sundae, making the pathogens they've bound to even more appetizing. Pathogen phagocytosis increases when an antibody is attached, and all that bad stuff becomes some phage's supper. And sometimes, an antibody can disrupt an essential bacterial cell process, like cell division, all on its lonesome, forcing the alien pathogen to self-destruct. Whether it's by making it a meal or sabotaging it from within, the immune system tries its best to clear out nasty invaders.
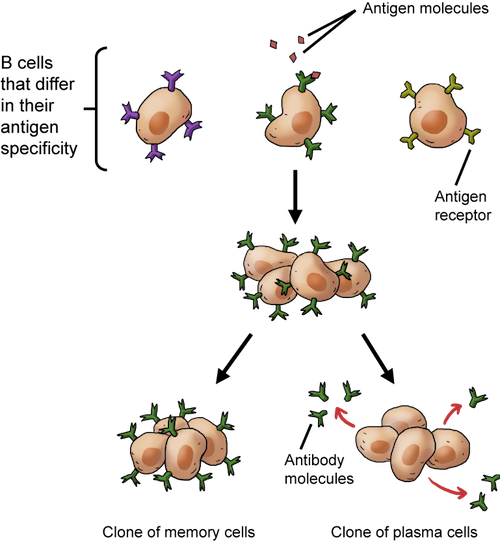
Clonal selection of B cells. When B cells bind to their particular antigen, they replicate and make cloned memory cells and cloned plasma cells that release antibodies.
In addition to making antibodies, B cells also produce daughter cells when they are activated. Some of the clones will become plasma effector cells that recognize the same antigens and produce the same antibodies. It's a true attack of the clones, since these replicas attack the current infection.
Other daughter cells carry grudges, and they are called memory cells. These cells stick around for a long time, remembering a particular infection. If there's another exposure to the same antigen sometime down the road, they trigger a larger and more rapid immune response, called a secondary response. In other words, they learn the tricks of the pathogen's trade the first time around, so the immune system can really bring the heat if the repeat pathogen has the guts to show its face again. This whole B cell cloning thing really ramps up the arsenal of antigen-recognizing manpower.
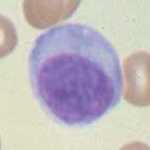
A zoomed-in picture of an actual effector plasma B cell. Image from here.
T Cells and the Cell-Mediated Immune Response
The other type of adaptive immune system in vertebrates is the cell-mediated immune response. Here, the body uses T cells to mount an attack on invading pathogens. Like B cells, T cells originate in the bone marrow as stem cells, but unlike their closely related alphabet-loving lymphocyte cousins, they travel to the thymus to mature—hence the "T" in T cells.These cells are highly specialized to fight off those sneaky pathogens that have made their way into host cells.
T cells work a bit differently than B cells; they only recognize antigens once the pathogen has already penetrated a cell. In the infected cell, intracellular proteins break the pathogen down into small parts. A group of proteins called the major histocompatibility complex (MHC) pushes these broken down pathogen parts (including the antigen) to the cell surface. When they express the antigen fragment and MHC, these cells are called antigen presenting cells. It's genius, we know.
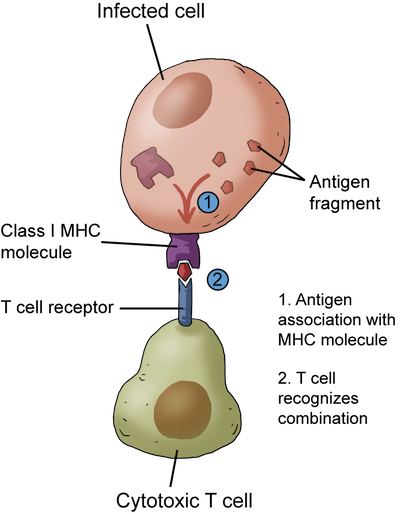
Antigen Presenting Cell. T cells recognize the antigen fragment and MHC complex on infected cells.
T cell receptors not only recognize certain snippets of antigen fragments poking through the cell membrane, but they also care about the specific class of MHC at the cell surface. It's an easy game of Hide-and-Seek for the T cells—attack the cells waving a red flag. End of game.
There are three different types of T cells, and each differs in the ways it regulates the adaptive immune response:
- Helper T cells
- Cytotoxic T cells
- Suppressor T cells.
Cytokines provide communication to B and T cells as well as phagocytes, and aid in their activation. There are hundreds of different cytokines. They can encourage immune cell growth, destroy infected cells, or enhance the innate immune system's inflammatory response.
Other helper T cell clones develop into memory cells. These are similar to the memory B cells, and "remember" a pathogen exposure to mount a quicker response the next time.
Cytotoxic T cells kill invading pathogens directly; they are the effectors of the cell-mediated immune response. They become active when they bind to an antigen-presenting cell (with the appropriate MHC) and when helper T cells release cytokines. Cytotoxic T cells release potent cell-destroying chemicals that can cause an infected cell to rupture, forcing those nasty invaders to fend for themselves and fight off circulating antibodies. It's not a fair match, and luckily our bodies usually reign victorious.
The suppressor T cells are the ones who do the checking, and the actually dampen the immune response by suppressing lymphocyte activation when things have gotten out of hand. It's also important to turn off the immune response when the body becomes healthy and all the troublemakers have been annihilated. All of the robbers are sitting with their hands above their heads, and the job is done.
Scientists aren't quite sure how these suppressor lymphocytes work. It's possible they suppress the immune response by recognizing a particular antigen and prevent other immune cells from attacking it. But they could also regulate how rapidly B and T cells replicate. Add it to your list of great scientific mysteries, along with the nature of the multiverse and who ate our last chocolate bonbon.
Immunity
Once activated, both B and T cells create antigen memory cells that help ward off future infections. Not only will memory cells mount a more rapid and robust secondary immune response the next time around, but they will also provide active immunity to the pathogen. Faster and stronger—what's not to like about that?Active immunity arises when an animal's own immune system protects the body from a pathogen. The memory cells produced by B and T cells provide active immunity against a particular exposure since they protect the body from harm. If your body naturally produces memory cells for immunity, it's called natural immunity. Vaccines are one way that we can induce natural immunity, by introducing small amounts of antigens without getting sick.
Vaccines stimulate the production of memory cells by tricking our bodies into thinking there's an infection. That means the immune system will attack this harmless pathogen and create antibodies and memory cells against it. Since the memory cells were made in response to an artificial infection, this is a type of artificial immunity. The long-term antibodies and memory cells protect us from the measles or this year's flu strain.
Another type of immunity, passive immunity, comes from an outside source and doesn't require the body's memory B and T cells. One of the most common forms of passive immunity is getting a dose of antibodies from some other source. The antibodies are produced in someone or something else and then transferred to you, and presto, you’re protected from harm. Antibodies being passed to a baby during pregnancy across the placenta, or transferred in breast milk, are actually the only line of defense against infection for infant mammals, at least until their bodies start making their own B and T cells.
Another example of passive immunity is antivenom. Sheep and horses are exposed to snake or spider venom, and then the antibodies that they produce are extracted. Then we can use that serum when we mistake a rattlesnake for a really cute belt. The tetanus vaccine is another example, which is an antisera that’s produced in horses.
Problems Arise
The immune system isn’t perfect. While the antigen/antibody relationship is a pretty specific one, our bodies can occasionally recognize something as an invading pathogen when it’s just a harmless bit of pet dander or a peanut. When we first encounter an allergen and its antigen, a primary immune response ensues, and the reaction (if any) will be pretty mild. When we encounter it again, we now have those memory cells that ramp up quickly, that can cause some pretty serious symptoms.In addition to allergies, our bodies can mount immune responses to other foreign substances, like blood or organs. Blood typing is extremely important when a person receives a transfusion, because antigens on the red blood cells will be seen as a pathogen by our immune systems. Phagocytes will attack red blood cells and present their antigens as a signal for other immune cells to mount up. If you're curious, you can read more about blood transfusions and immune responses here.